Exoplanets and planet formation
At Lund Observatory we study planets orbiting distant star – exoplanets. We are particularly interested in the formation of exoplanets in gaseous protoplanetary discs, the stability and survivability of planetary systems and exoplanetary atmospheres. Listed bellow are a few of our ongoing projects.
Observing exoplanet atmospheres with state-of-the-art spectrographs
Researchers include: Jens Hoejimakers, Bibiana Prinoth, Nicholas Borsato, Brian Thorsbro
State-of-the-art spectrographs have the ability to observe the chemical signatures of the atmospheres of hot, close-in exoplanets. Transit and day-side emission spectra contain the faint signatures of atoms and molecules, which we use to understand the chemistry, structure and dynamics of the atmosphere. In addition, adaptive-optics assisted slit or integral-field spectroscopy can be used to resolve hot, young planets in wide orbits, providing inputs to planet formation models.
In Lund, we focus on developing spectroscopic methods and models to observe and understand the atmospheres of the hottest gas giant exoplanets exoplanets. With new, powerful spectroscopic facilities on the horizon (JWST, ELT, Ariel, etc.), these insights will help us to study cooler, smaller planets and search their spectra for signatures of habitability and life.
White dwarf planetary systems
Researchers include: Alexander Mustill, Judith Korth
What are extra-Solar planets made of? To answer this, we turn to the dead stars known as white dwarfs. Many have heavy elements detected spectroscopically in their atmospheres. These are delivered by asteroids scattered close to the white dwarf that break up and deposit their material in its atmosphere. Understanding this process is the only means we have of determining the bulk elemental compositions of extra-Solar asteroids and planets.
Planet formation and evolution of protoplanetary discs
Researchers include: Anders Johansen, Katrin Ros, Johan Appelgren
Planets form in protoplanetary discs around young stars as dust grains collide and stick together to form larger and larger bodies. Protoplanetary discs are observed around most young stars. They play two important roles: they are birth sites of planets and they feed gas to the star via accretion.
At Lund Observatory, we study how the discs form and evolve, turbulence in the disc, and the formation of planetesimals.
Turbulence
The stresses associated with turbulent gas motion can transport angular momentum outwards and mass inwards. Our best candidate for driving turbulence in protoplanetary discs is the magnetorotational instability. Keplerian gas flow is in itself stable and will not transition to turbulence, despite of the free energy in the shear. The presence of a weak magnetic field changes the situation entirely and constitutes a robust path to turbulence in the regions where the disc is sufficiently ionised.
We study the details of protoplanetary disc turbulence caused by the magnetorotational instability. We use the Pencil Code to simulate the magnetohydrodynamics of gas in protoplanetary discs.
Planetesimal formation
The formation of km-sized planetesimals from smaller cm-dm sized particles has faced difficulties in the traditional coagulation scenario. Such particles do not stick well and very quickly drift towards the star to sublimate in the inner nebula. Pressure bumps may concentrate particles enough that the particle component contracts gravitationally to form planetesimals. In regions where the ionisation fraction is too low to support magnetic turbulence, the streaming instability constitutes another possible path to planetesimals.
Planetesimal formation takes place in a complex environment of turbulent gas interacting via drag forces with particles of many sizes. The streaming instability thrives in the systematic relative motion of gas and particles and leads to spontaneous clumping of particles. The gravity from the star is partially compensated by the radial pressure gradient of the gas, causing gas to orbit at a slightly slower speed than Keplerian. Clumping is initiated as initially very low amplitude particle overdensities accelerate the gas towards the Keplerian speed, hence reducing the local head-wind on the particles, which in turn slows the radial drift of the particles. Drifting particles pile up where the head-wind is slower, causing exponential growth of the particle density as the particles continue to increase their drag force influence on the gas.
The process can be compared to how bicycle riders and migrating geese travel together to protect themselves from the headwind of the atmosphere.
The particle filaments become very dense, up to several thousand times the local gas density. The collective gravity of all the particles is high enough to initiate a gravitational collapse to form planetesimals with a characteristic radius of 500 km. This is comparable to the dwarf planet Ceres in the asteroid belt.
The particle filaments become very dense, up to several thousand times the local gas density. The collective gravity of all the particles is high enough to initiate a gravitational collapse to form planetesimals with a characteristic radius of 500 km. This is comparable to the dwarf planet Ceres in the asteroid belt.
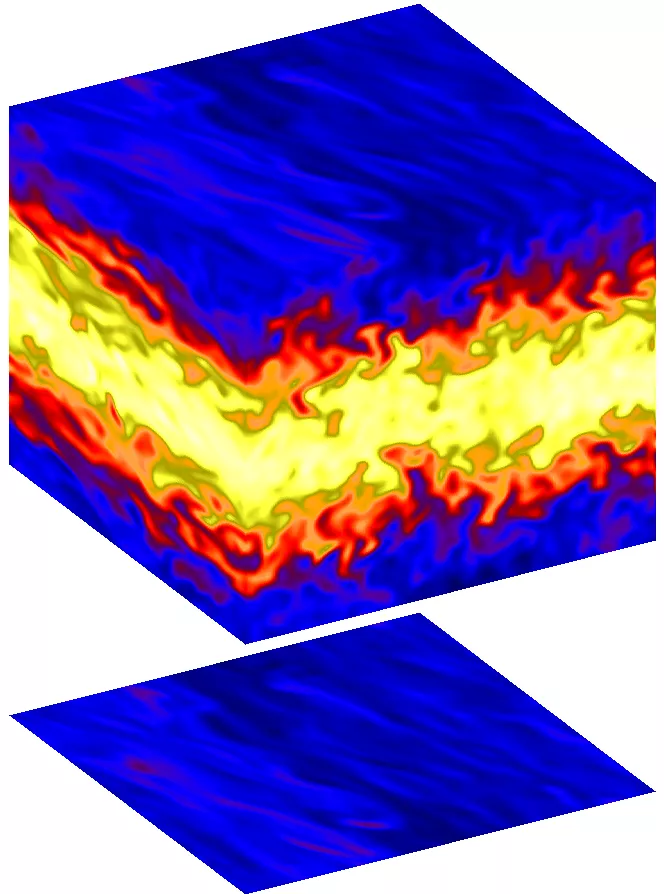